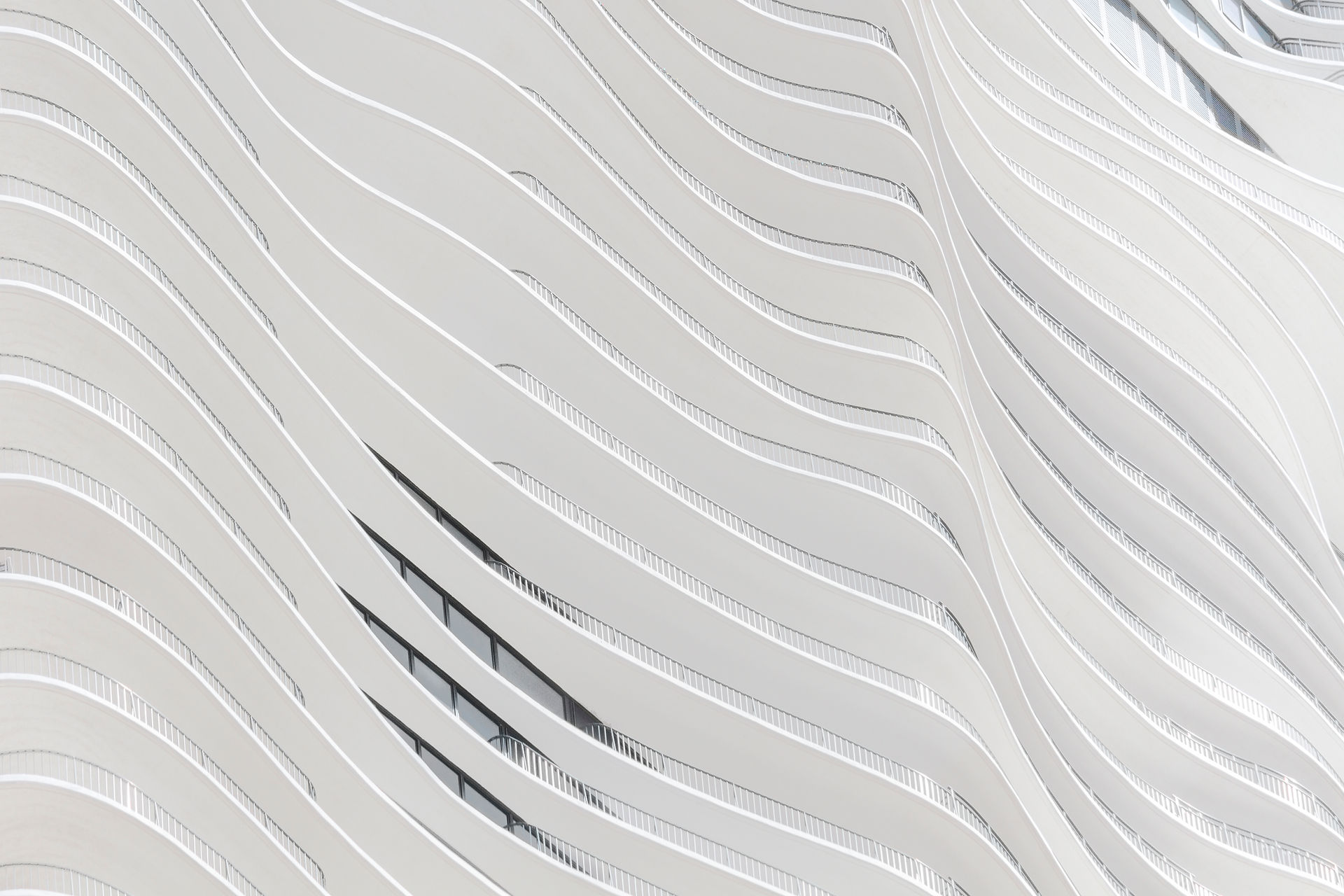
Research in the Allingham laboratory focuses on dynamic protein complexes that generate pico newton-scale forces that add up to produce changes in cell shape and whole-cell movement. Our primary interests are kinesin motor proteins, microtubules, and actin filaments. Our goal is to understand these proteins' force-generating mechanisms by determining the 3D shapes of the complexes they form by using X-ray crystallography and electron microscopy. We also use biochemical analyses, molecular genetics, cell biology, and fluorescence microscopy to visualize and measure structural changes in these complexes and the actions that result.

Design and development of actin-targeting compounds
Cancer was responsible for approximately 10 million deaths in 2018. Cancer metastasis accounts for over ninety percent of these deaths and is one of the most significant challenges in cancer treatment because, in many patients, metastasis has already occurred by the time they are diagnosed with primary cancer. Current cancer therapies fail to prevent metastasis in many patients with advanced cancers. With funding from CIHR, NSERC, and the New Frontiers in Research Fund, Allingham lab scientists and our collaborators are developing simplified analogs of actin-targeting natural products that inhibit cancer cell metastasis (e.g., Mycalolide B). We are also exploring strategies for targeting these analogs to cancer cells, beginning with conjugation to clinical-grade oncology antibodies. At the same time, we have initiated studies to define the impact of multivalency of these synthetic toxins toward enhancing their antimetastatic activities.
Roles and regulation of kinesins in pathogenic fungi
Fungi are significant and increasing mediators of pathogenesis, causing severe challenges medically and economically. Candida albicans is the most prevalent cause of fungal infections in people and can cause life-threatening systemic infections if our immune defenses are compromised. A battery of fitness attributes enables drug resistance of this fungus, most of which arise by the rapid generation of genetic diversity within a population as a means of adaptation. Research has shown that aneuploidy (an abnormal number of chromosomes) accounts for much of this diversity. This condition often arises from changes in components of the chromosome segregation machinery. Allingham lab researchers have discovered that some of these changes could be induced or enabled by altered activity of kinesin motor proteins that regulate the cell's chromosome segregation apparatus – the mitotic spindle.
The main goal of this CIHR-funded research program is to understand how kinesins regulate the structure and function of the mitotic spindle in the human fungal pathogen Candida albicans. Our specific aims are to: (1) determine the three-dimensional structures of kinesins that drive mitotic spindle assembly and chromosome aggregation in C. albicans, (2) to identify which of these kinesins are critical parts of stress-adaption mechanisms that promote mitotic errors leading to beneficial aneuploidy, and (3) to identify kinesin binding partners that regulate kinesin function under stress.
Molecular mechanisms of kinesins that control cytoskeleton structure
Eukaryotic cells contain a dense array of microtubules and actin filaments that serve as the cell's cytoskeleton and as substrates against which molecular motor proteins generate force. Microtubules are used for intracellular transport, cell motility, cell signaling, and chromosome segregation. Actin filaments control the cell cortex's architectures and mediate cell motility, cell division, and endocytosis. When cells transition through each cell cycle, or change morphology, they disassemble these arrays and reconstruct them into new configurations according to their functions. This process is also highly regulated in cells by many microtubule- and actin-binding proteins and cofactors, a major one being kinesins.
Understanding the molecular actions of kinesins on microtubules and actin in cells is challenging, especially when multiple kinesin types can be operating simultaneously, or when numerous activities coexist within a single kinesin motor. Therefore, much of our knowledge of these cytoskeleton regulators requires in vitro biochemical and structural studies. Our short-term aims of our NSERC-funded research are to: (1) determine how subtle tweaks and edits within the amino acid sequence of kinesin-3, kinesin-8, and kinesin-13 family members has diversified their mechanism of cytoskeleton regulation, and to (2) construct structural models of each kinesin’s mechanism that explain the fundamental differences in their cellular functions. Our long-term aim is to understand how unique segments of amino acids from kinesins, and other cytoskeleton regulatory systems, can be recombined to create new biomolecular machines that work with engineered cytoskeleton tracks.